In my opinion, the Space Shuttle was hands down one of the coolest and most impressive feats of engineering that the United States ever designed. Something about the collaboration of sheer size and power alongside the delicate and intricate control of the vessel amazes me to this day. But, not everyone saw the Space Shuttle as a success. In reality, it was actually quite the failure.
When the Space Shuttle was first developed, it actually had similar goals to the SpaceX Falcon 9. It was meant to be reusable, with two detachable liquid fuel boosters, a smaller cargo hold, heat protection similar to the X-15 spaceplane, and it was supposed to be cheap to operate with a quick mission turnaround. In short this did not happen. Thinking historically, the Space Shuttle program was beginning during the 1970’s. This is when the Vietnam War was getting into full swing and many of the United States’ resources were being shifted towards the war effort. This left NASA with a much smaller budget than they had anticipated, just 5 billion dollars to be exact.

5 billion dollars may seem like a lot of money to the average Joe, but 5 billion dollars is an incredibly tight budget for the development of an entirely new Spacecraft. In a study by a firm called Mathematica, it was estimated that the development of the reusable boosters themselves would cost upwards of 10 billion dollars. In order to get more funding, NASA reached out to the U.S.A.F. The Air Force was more than happy to contribute to the funding of the Space Shuttle, but they demanded jurisdiction over the size and capacity of the cargo hold on the Shuttle. The Air Force required a much larger and heavier cargo hold than before, which pushed NASA to make even more changes.
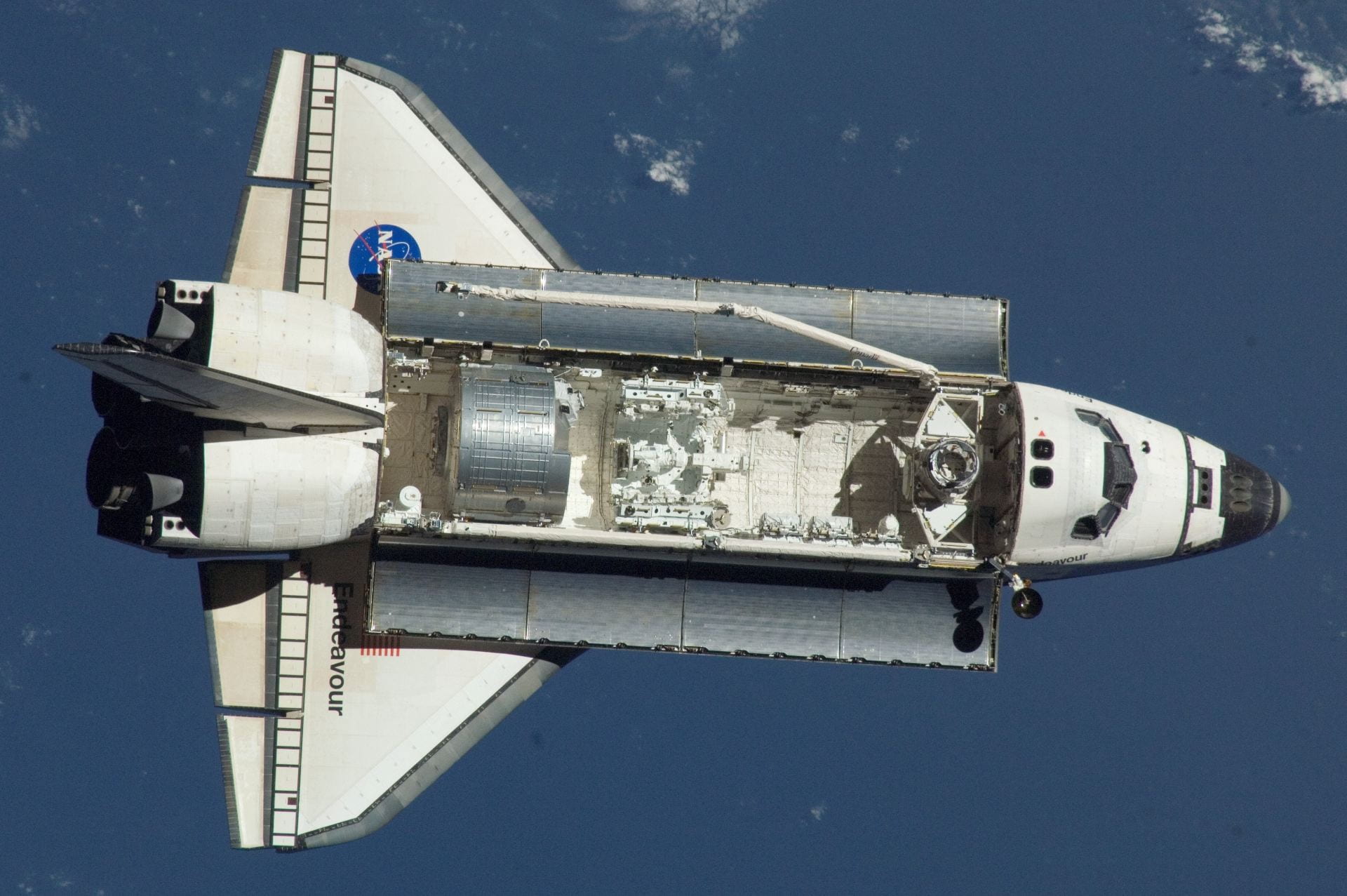
Since the spacecraft needed to be so much larger, heat dispersion techniques like on the X-15 were impossible. NASA had to resort to ceramic heat tiles. There were hundreds of these tiles that needed to be fastened to the outside of the Shuttle. This caused the Shuttle to weigh much more than beforehand, so NASA had to go back to the drawing board on what type of boosters they could use. Because of the strict budget restraints, NASA could no longer use the more expensive and reusable liquid fueled rockets. They had to switch over to solid fuel rockets which were cheaper, but much more powerful.


At this point, NASA had a Space Shuttle that was far from their original design. It used different boosters, was much larger, had heat protection tiles, and was built with concerningly tight monetary budgets. All of the changes and corner cutting led to disaster. The Space Shuttle had two major catastrophes, cost more than anticipated to operate, and took ages to get back in shape for another mission.
The first disaster among the Space Shuttles was the Challenger disaster of 1986. This happened due to the O-rings in the solid engines malfunctioning. The launch date was cooler in temperature than the other Space Shuttle launches. The lower temperature shrunk the O-rings in the booster leading to an oxygen leak and, soon after, disaster. The entire disaster could have been prevented without the need for the solid boosters. This was one of the risks that the space program understood when choosing the solid boosters, but had no choice but to accept with the budget constraints.

The second disaster was the Columbia disaster of 2003. This disaster occurred due to the problematic heat dissipation tiles fitted to the outside of the Shuttle. When the vessel was reentering the atmosphere, some of the heat protection tiles ripped off of the Shuttle. The exposure of the internal space Shuttle caused a rapid increase in heat, explosion, and ultimately disintegration. This was another example of how the design constraints caused the STS to fail. If the Shuttle had been designed as it originally was supposed to be, the Shuttle would not have used the risky heat tiles.

Finally, the Shuttle was a financial disaster. Each mission cost the Shuttle more than 12 months of repairs. This was much longer than anticipated, especially since the Shuttle was designed to be quickly returned to space. In addition, the Shuttle cost more than anticipated to operate. So ultimately the STS program was a big use of the Nation’s money.
All in all though, the STS program was one of the Nation’s proudest eras. The STS launched hundreds of successful missions and paved the way for more space travel in the future. The Shuttle program influenced people across the nation and gained support for the exploration of the unknown!