This post is the fifth in a short blog series called “Know your Insect”. The images and descriptions are written by Entomology graduate students enrolled in a seminar of the same name.
Like most other animals, insects have a central nervous system that helps them sense and respond to their environment. The central nervous system of insects (along with the rest of the arthropods and many other invertebrates) is called the ventral nerve cord, and it consists of a brain, a part underneath the brain called the sub-esophageal ganglion, and a cord that runs along the length of their body. This cord is similar to our spinal cord except that it runs down the front of their body instead of the back. So the quickest way to get to the nerve cord of a cockroach (or any insect) is to cut open its belly!
Structure of Ventral Nerve Cord
If you actually did cut open a cockroach and take out its nerve cord, it would look a little like a string of beads. The “beads” are clusters of nerve cells called ganglia, and the string-like connections between them are called connectives. The ganglia and connectives can be arranged different ways in different insects. The very first insects had their ganglia roughly evenly spaced along the nerve cord, with one ganglion innervating (sending signals to) each body segment, including 3 thoracic segments and 8 abdominal segments (Niven et al., 2008). Some insects like bristletails still have nerve cords like this, but in many other insects some of the ganglia are closer together than others, and they may even fuse to form larger ganglia.
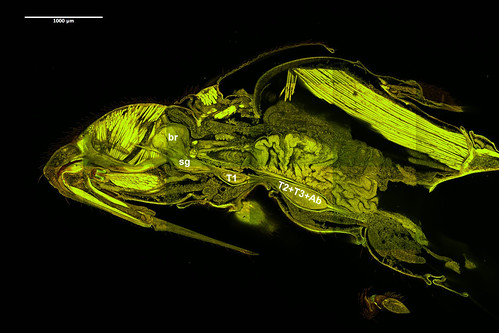
And you don’t even have to look at distantly related insects to see differences in their nerve cord structure; some closely related species of stingless bees have very different arrangements of their ganglia (Wille 1961), and there can even be differences between males and females of the same species (d’Herculais 1881) or between workers and queens (which are both female but develop differently) (Niven et al., 2008).
Function and Evolution
It’s not clear what all this diversity is for, but a general theory is that the insect nerve cord is arranged to minimize total wiring cost, balancing speed, space, and energy (Niven et al., 2008). Also, ganglia that are closer together can send signals to each other more quickly, and scientists think that some insects may benefit more from signaling quickly to adjacent neurons along the nerve cord, whereas others might prioritize signals sent to peripheral neurons (those that directly control motor movement) (Niven et al., 2008). Variation in life history traits could tip the balance one way or the other—for example, female conopid flies have an abdominal ganglion very far back in their bodies, whereas in males it is much farther forward. It is thought that this different arrangement could have evolved because females need fine control of complex muscles for egg-laying, whereas males do not (Streiff 1906).
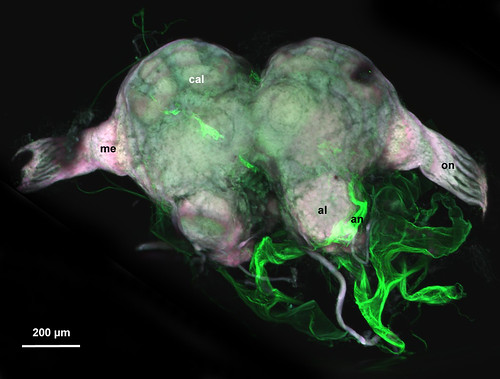
The insect brain
While all ganglia on the ventral nerve cord contribute to organizing, managing, and synchronizing information that ultimately directs the insect’s behavior, the supraesophaegal ganglion (or brain) is the one that has been studied the most. And actually, the brain is made up of three fused ganglia that have different functions. The protocerebrum is the largest and most complex ganglion and includes the optic lobes (ol: Figs 2, 3) for vision and the mushroom bodies (cal: Figs 2, 3) which are involved with learning and memory and also processes olfactory (smell) information. The deutocerebrum includes the antennal lobes (me: Figs 2, 3), which receive information from the insect’s antennae. The tritocerebrum connects the brain to the rest of the nerve cord and also innervates some of the insect’s mouthparts.
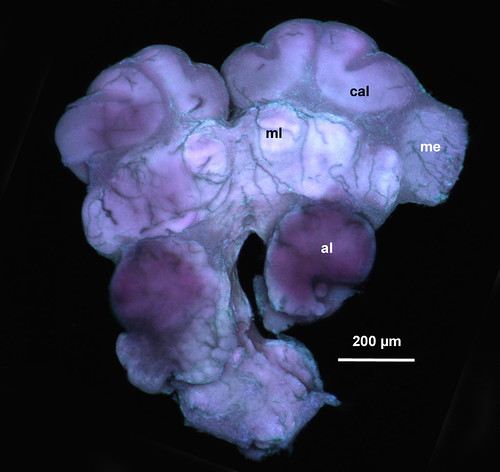
Studying the insect brain
Early research on the insect nervous system included ablation experiments, where researchers would take out part of the brain (or cut off the whole head!) to see how this would affect the insect’s behavior. These experiments taught us a lot about how the insect nervous system works. For example, Donald Wilson found out that central pattern generators for locust flight originate in the thoracic ganglia, meaning that headless locusts can still fly (Wilson 1961a), and Kenneth Roeder found out that headless cockroaches can learn to avoid an electric shock (Roeder et al., 1960).
Nowadays, much of the research on insect brains is done with the help of advanced microscopy techniques such as CLSM (confocal laser scanning microscopy). For example, researchers have used these techniques to show that ants’ brain morphology can change over their lifetime as they age and switch tasks in the nest (Gronenberg et al 1996), and that whether ants are large or tiny, the density of their synapses (so how tightly packed their neurons are) in certain brain regions is similar (Groh et al 2014).

My research: zombie ants
Part of my research involves using CLSM to study brain morphology of ants. Specifically, I am trying to find out if a behavior-controlling parasite changes the way an ant’s brain looks under the microscope. I will take images of healthy ant brains and compare these to brains of ants infected with the fungal parasite, Ophiocordyceps unilateralis. This fungus causes infected ants to leave their colony and bite onto the underside of a leaf or twig before they die. This behavior is adaptive for the fungus because being above the ground and close to ant foraging trails allows it to grow out of the ant’s head and shoot spores to the ground below where it will infect other ants (Andersen et al. 2009; Hughes et al. 2011). I will also use a fungus that kills the ants but does not manipulate them, to find out what is special about the brain of a manipulated ant compared to one that is just sick. From this research, I hope to eventually understand how the fungus controls the ant’s behavior. And if we understand how a brain can be manipulated, then we can better understand how normal brains work.